Peto’s Paradox: Why Larger Animals Aren't More Prone to Cancer
Larger animals have more cells, which means they should have a higher incidence of cancerous tumors. However, they don’t—elephants and whales, the largest animals in the kingdom have extremely low rates of cancer.
Reading Time: 4 minutes
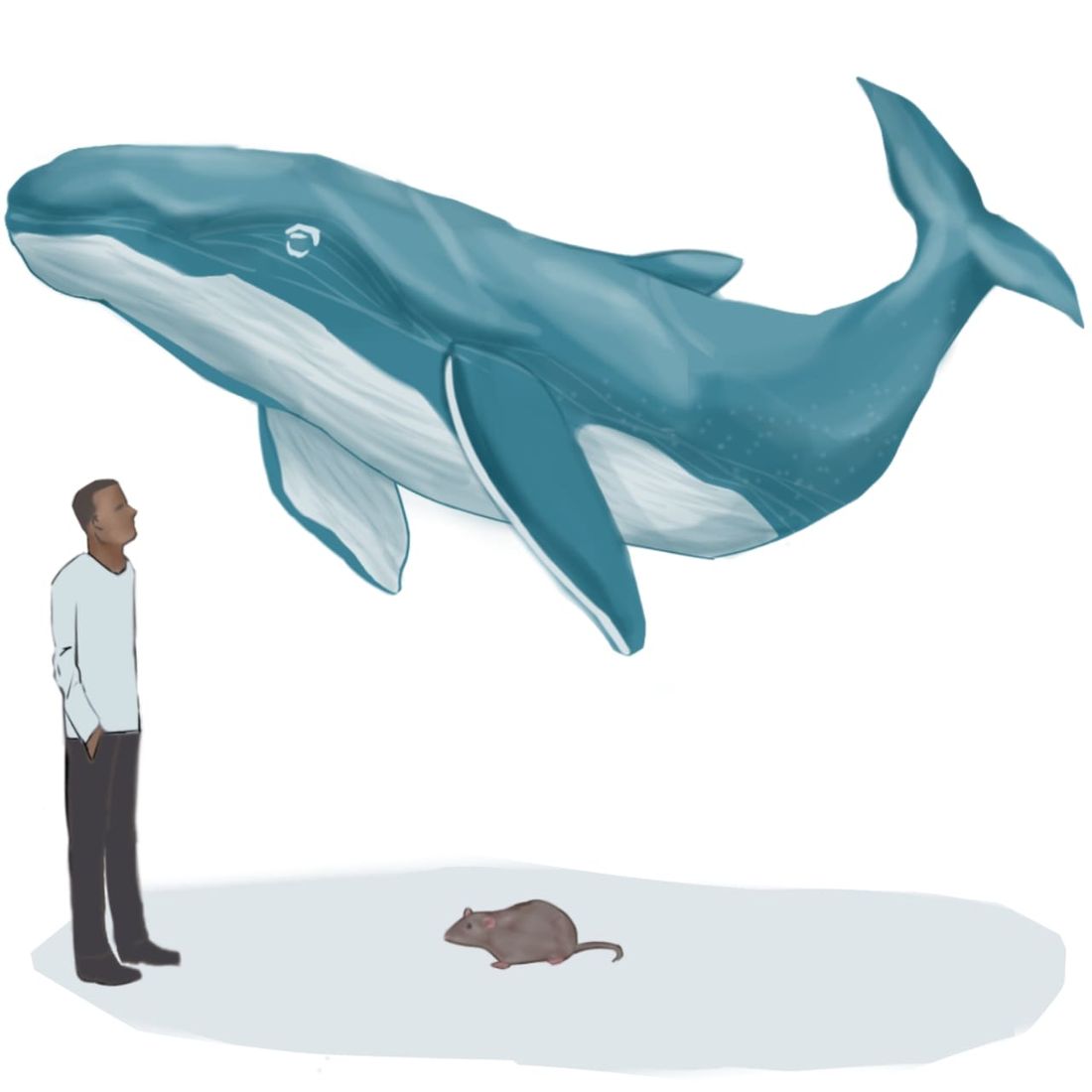
For over a century, cancer has been the greatest puzzle that scientists and physicians have tried to solve. It claims nearly 10 million people worldwide annually and affects hundreds of millions more. Cancer is a disease characterized by the uncontrolled growth of abnormal, mutated cells. Under normal conditions, cell division is strictly regulated by checkpoints during mitosis—the process of cell replication—and tumor suppressor genes. However, genetic mutations that affect these pathways may arise, ultimately leading to cancer. In hopes of finding a cure for the deadly disease in humans, some scientists have wondered how other animals deal with cancer
In 1977, British epidemiologist Richard Peto hypothesized that the more cells an organism has, the higher the chances are for a cell to genetically mutate and grow uncontrollably. In other words, smaller organisms like rats and birds would be less likely to die from cancer than larger animals like whales and elephants because there were less chances that one of their cells would mutate. However, the contrary was found to be true. According to the National Institute of Health, “elephants remain cancer resistant, with an estimated cancer mortality of 4.81 percent… compared with humans, who have 11 to 25 percent cancer mortality.” Similarly, cetaceans like whales, dolphins, and porpoises have cancer rates of 0.7 to two percent. Since Peto was the first to notice a discrepancy between tumor incidence and animal size, the term for such a relationship was coined by the scientific community as “Peto’s Paradox.”
Over the past few decades, scientists have made numerous theories to explain the lack of correlation between body size and cancer risk. The most-accepted explanation is the relatively high counts of p53 genes in larger animals. Known as the “guardian of the genome,” p53 is a tumor suppressor gene known to prevent abnormal cell growth. P21, a protein activated by p53, blocks cyclin-cDK complexes—proteins that progress the cell cycle. This halts cell division, giving the cell more time to repair its DNA before it replicates. However, cell damage is beyond repair in some instances. If this is the case, p53 goes on to activate BAX, PUMA, and NOXA—a group of pro-apoptotic genes which trigger apoptosis, or programmed cell death. While whales have over 20 copies of p53, humans only have one. Furthermore, each of the 40 alleles of this gene have slight variations that cover a broader range of cancers. The reason for such variation is likely due to a strong evolutionary pressure for cancer-resistant whales. The whales with more tumor-suppressor genes survived significantly longer than those without as many, causing the cancer-resistant coding genes to be present in greater concentration in later generations. However, smaller animals like squirrels and rodents often die from predation long before cancer sets in, and thus high cancer-resistance wasn’t selected for.
Due to the slower rates of cell division caused by p53 in cetaceans and elephants there is a lower incidence of cancerous cells in these animals. However, there are potential downsides to having so many copies of this cancer-fighting gene. Animals with lower rates of cell division tend to produce not as many sperm cells. As a result, they tend to be less fertile and produce less offspring than those without as many copies of this gene. Consequently, only animals with the highest selective pressure for tumor-suppression have managed to evolve in this direction. More intermediate-sized mammals like humans unfortunately would have not acquired these more extreme cancer resistant traits.
A more unlikely theory involves the instability and high rate of mutation in cancerous cells. Arizona State University professor John D. Nagy hypothesized that natural selection favors aggressive tumors. One of the cancerous cells could mutate again to form a tumor on top of an existing tumor, starving and killing the original cancer cells.
However, these hyper-tumors are unlikely to be found in smaller animals. This is because there is a positive correlation between the size of an animal and the time it takes for a tumor to become deadly in that organism. If, for example, two gram tumors were to exist, each on a rodent, a human, and a whale, the mouse’s tumor would make up approximately 10 percent of its body weight. On the other hand, it would make up less than 0.002 percent of a human’s body weight and less than 0.000002 percent of a whale’s. Even though tumors grow at the same rate in both organisms, they affect different-sized organisms very differently. In the rare case smaller mammals get cancer, they end up dying of it long before another tumor can grow on top of it.
Thankfully, the ways larger animals combat cancer have proven valuable in the field of oncology. Throughout the years, scientists have conducted many experimental trials that target faulty tumor suppressor genes. In 1996, Jack Roth was the first to attempt p53 gene therapy in humans. He used a viral vector expressing the p53 protein to treat several patients with lung cancer. Remarkably, tumor regression was found in three patients, and tumor growth stabilized in the other six. Furthermore, programmed cell death was found to affect cancer cells more severely than healthy cells, making treatment somewhat targeted. In more recent news, numerous clinical trials involving p53-based vaccines have begun underway. Gene therapy companies like Advexin continue to inch closer towards approval by the FDA.
Ultimately, researching the mechanisms of cancer-resistant animals provides valuable insights as to how nature has already “beat” cancer. Solving this puzzling paradox could both deepen our understanding of evolutionary biology and lead to breakthroughs for the treatment of cancer. With recent advances in the field of genetic engineering, it has become more possible than ever to incorporate tumor suppressor genes into our own genome. At a school like Stuyvesant, where innovation and problem solving run deep, Peto’s Paradox offers us a reminder that not everything follows the rules we expect. Just like Mother Nature, we can tackle big problems by questioning assumptions and thinking outside the box.